
It's helped by the fact that bacterial genomes aren't completely random, and can usually be seperated into 'core' genes and 'accessory' genes. Core genes are most useful to taxonomists as they show what the bacterial species actually is, and where it sits within molecular phylogeny. The accessory genes are more interesting to bacteriologists as the play a more significant role in phenotypic differences and determin what the bacteria do. Paradoxically, the accessory genes are also the ones used most in adaption (think of antibiotic resistance) and are therefore more likely to be evolutionarily selected for or against. These accessory genes are often found in specific 'hypervariable' regions of the genome called genomic islands.
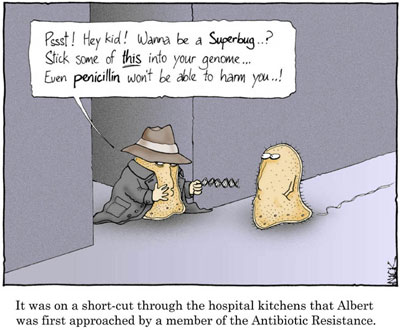
The increase in second-generation sequencing technologies opened up the ability for large scale comparitive-genomics studies; essentially sequencing huge numbers of bacterial genomes and then comparing them all to each other. Back in the day, population studies were done using an approach known as MultiLocus Sequence Typing (MLST) - which took seven core genes and sequenced those for each bacteria. Nowadays you can sequences all the parts of the genome you need in hundreds of different strains.
As with most new microbiology technologies, these new techniques are initially being applied to human pathogens, as human pathogen research is (rightly) where most of the money is. Using wholescale sequencing technologies on pathogens such as Clostridium difficile which has high levels of antibiotic resistance. The grand aim is to try and understand both the extent and the distribution of natural genomic variation between one bacterial species. This could help to understand what roles are played by bacterial migrations, recombinations (switching DNA around), active selection and drift in the spread of antibiotic resistance.
As with most new microbiology technologies, these new techniques are initially being applied to human pathogens, as human pathogen research is (rightly) where most of the money is. Using wholescale sequencing technologies on pathogens such as Clostridium difficile which has high levels of antibiotic resistance. The grand aim is to try and understand both the extent and the distribution of natural genomic variation between one bacterial species. This could help to understand what roles are played by bacterial migrations, recombinations (switching DNA around), active selection and drift in the spread of antibiotic resistance.
C. difficile is a very genetically diverse species which has been evolving for the last 1.8 million years. As each bacteria is likely to replicate at least once a day, this allows for a huge amount of change and genetic variation. It might be suspected that the virulent species is formed from a single offshoot, one bacteria that became virulent and then passed that ability onto its offspring but instead it looks like virulence arose in many different lineages, most likely through horizontal gene transfer. This means that each different virulent strain is likely to show a relatively large amount of variation in the rest of its genome.
It isn't just small bits of DNA on separate plasmids that C. difficile can exchange, they can also carry out homologous recombination, a process by which whole sections of the bacterial genome can be cut out and shared between related species. This process is aided by the presence of 'mobile elements' within the genome, i.e pieces of DNA which are particularly good at jumping around and splicing themselves in and out of bacterial chromosomes. It turns out that the C. difficile genome contains lots of mobile elements.
From a bacteriologists point of view this is a fascinating example of just how variable a single strain of bacterial species can be. From a medical viewpoint it's more worrying. The ability of highly virulent bacteria to chop out large portions of their genome and pass them onto other, potentially non-virulent strains could help to spread not just antibiotic resistance, but also other tricks like biofilm formation and different enzymes which help the bacteria to cope with antibiotic challenges.
Not only that but it means that every gene in the genome has been tried and tested by many different strains in many different conditions. They aren't just good genes for the bacteria to be hanging onto. They're the best.
---
8 comments:
Love the cartoon!
Not only is this shuttling of genes worrying - it is also humbling! In terms of adaptive potential, bacteria really put us to shame. Evolution is their game and we get to play along sometimes :).
@Sue: Thanks! It's one of my favourite, and I've been looking for an excuse to use it for ages.
@Lucas: I was completely blown when I found out they could chop and change whole swathes of genome. With so much adaptive potential no wonder they can just expand into any niche they find.
The diversity in bacteria is truly mind-blowing. Every human being must be their own unique bacterial eco-system, I'm thinking.
The difference you point out between pro- and eukaryotic evolution suggests that eukaryotic sexual repoduction is a mechanism to generate genetic variants among offspring, a necessity as generation time increases and the ability to swap genes decreases.
@yogi: Pretty much yes! Luckily most of the human ecosystem will be commensal and non-dangerous bacteria rather than pathogens.
@JE: That's pretty much how I think of it! Bacteria can swap around genes as much as they like, but in eukaryotes the genes are trapped in the nucleus. Sexual reproduction is therefore necessary in order to get any kind of genetic variation.
Two questions:
1. Anyone know if any research is being done into the genes that impart antibiotic resistance to the bacteria? It would seem that if there is a gene that is being exchanged between bacterial species that made them resistant to antibiotics, it would be worth researching that gene more than the organisms as a whole, no?
2. How does the genetic variability within a species of bacteria compare to the variability of something like humans?
@Casey:
1-YES! There is *loads* of research going into antibiotic resistance, the first step anyone does is find the gene. I think now in almost all cases of antibiotic resistance it's known which gene causes it, and what that gene does. Usually it's just one gene as well, that is passed between bacteria.
2-Bacterial 'species' are hard to define, but generally there is a lot of variability between them, as the genomes are smaller, and they can pick up DNA from unrelated species. With humans on the other hand, the genome is much, much larger. The genetic variability between humans is being worked on, now we have a few more human genomes it looks to be larger than people first thought, but bacteria are far more variable.
Post a Comment